Your cart is currently empty!
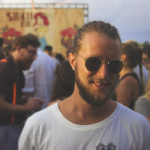
Williams Brown
Lorem ipsum dolor sit amet, consectetur adipisicing elit. Dolor, alias aspernatur quam voluptates sint, dolore doloribus voluptas labore temporibus earum eveniet, reiciendis.
Latest Posts
- Democrat at center of Kilmar Abrego Garcia saga responds to angel mom Patty Morin
- Secret Service Director Sean Curran offers behind-the-scenes look at what it takes to become an agent
- Girl, 14, killed by lion in Kenya
- Calls for US action amid growing violence against Christians in Nigeria
- Pope wishes worshippers Happy Easter after serious illness
Categories
Archive
Tags
Social Links
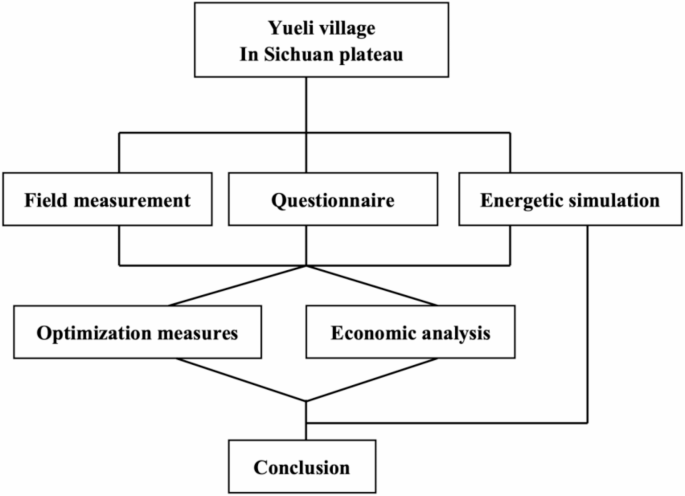
Parameter optimization and simulation of Building envelope structure
Using the typical meteorological data of Aba Prefecture in the Chinese Standard Weather Data (CSWD) of DesignBuilder, the indoor thermal environment of the building was simulated in July of the test period; in the meantime, The indoor temperature is set to 18 °C. Except for the bedroom and living room where people live, the rest of the room does not require a heat load. According to commonly used building materials combined with the General Code for Building Energy Conservation and Renewable Energy Utilization GB55015-2021, when the building is no more than 3 floors, the heat transfer coefficient limit for roof is 0.2 W/(m2·K), for exterior wall is 0.3 W/(m2·K), for interior wall is 1.5 W/(m2·K), and for floor is 1.5 W/(m2·K). Combined with the calculation parameters of the thermal physical properties of building materials specified in the “Code for thermal design of civil buildings” GB50176–2016. The main materials of the optimized simulated envelope structure, and their thermal conductivity, specific heat capacity, dry density, and other interrelating parameters were determined. The thermal parameters of external walls, floor and roof in the software are given in the Tables 7, 8 and 9.
Building plane function optimization
The existing traditional houses have poor ventilation and lighting, and the indoor relative humidity is high. The architectural design can be explained in Fig. 13a: the entrance door of the living room and the adjacent room do not form a ventilation hall. Secondly, there is a kitchen at the back of the living room, and there is no external wall of the living room with direct windows, resulting in poor indoor ventilation and poor air circulation, and even if the moisture is volatilized, it will remain indoors, resulting in high indoor relative humidity, so indoor ventilation and dehumidification treatment should be carried out to obtain better indoor thermal comfort; In addition, there is no moisture-proof structural layer on the indoor floor of the traditional house, and the moisture in the foundation soil penetrates upward, resulting in high indoor humidity, and the outer envelope structure such as the exterior wall and roof does not have a waterproof structural layer, resulting in the retention of rainwater and so on, resulting in a high relative humidity of the house, so the waterproof and moisture-proof structural layer should also be done in the enclosure structure part, so as to effectively eliminate the phenomenon of ground moisture return and wall mildew in the traditional house.
The optimized architectural plan design is shown in Fig. 13b, the living room space is expanded, the original kitchen on the north side is adjusted to the west side, the south-facing windows are added, the south window-to-wall ratio is increased, and the north windows of the living room form a penetrating air, which improves air circulation, improves ventilation and lighting, and at the same time considers the local cold climate conditions, and reduces the window opening area on the north, east and west sides; Before the renovation, people had to pass through the living room to enter and exit the bedroom, kitchen and other rooms, resulting in mutual interference between flow lines and line of sight. After the optimization, the functional zoning of the building plan is clear, and the indoor toilet that is not available in the existing traditional houses is added to prevent residents from going to the toilet outdoors in the cold winter. The bedroom, kitchen, bathroom, and other private and functional spaces are separated from the living room and other public activity spaces, and the indoor circulation line is re-planned to make the activities of the personnel between different rooms smoother and more efficient, thereby avoiding streamline crossover and line of sight interference. The reformed plan ensures that each room has good natural lighting and ventilation. The indoor environment was improved by adjusting the position and size of windows, separating the roof and wall, and adding side windows, as shown in Fig. 13c. The façade of the optimized traditional houses is simple and rugged, retaining the unique architectural style and cultural connotation of the local ethnic minorities, and the plan focuses on improving the independence and efficiency of the space while maintaining visual comfort and privacy.
Potential implementation challenges and solutions
While these optimization measures are technically possible, they may still face a variety of potential economic, cultural, technological, and social challenges in practice.
Economic challenges: for example, adding a waterproof and moisture-resistant structural layer to the envelope requires a corresponding financial investment, which can be an unbearable burden for residents with limited financial resources. It is necessary to actively apply for national poverty alleviation funds or local governments can set up special funds to support the implementation of these optimization measures. At the same time, the participation of private enterprises and social organizations should be encouraged to reduce the economic pressure on residents.
Cultural challenges: optimization measures may not be in harmony with the architectural style of traditional houses, which requires that the architectural style and cultural characteristics of local traditional houses be fully considered when designing optimization measures to ensure that the optimized houses maintain the local architectural style and maintain harmony with the surrounding environment.
Technical challenges: in the traditional houses of ethnic minorities, the construction difficulties brought about by the above-mentioned optimization measures may be greater, relying on the experience left by local craftsmen, villagers and ancestors, traditional customs and culture, and personal intuition, and the lack of scientific and standardized professional knowledge, which can easily lead to potential safety hazards and quality problems. A professional construction team should be introduced to use advanced technical means to deal with complex construction problems. At the same time, communicate closely with residents to understand their needs and expectations to ensure a smooth construction process.
Social challenges: some residents may be sceptical of the optimization measures and are unwilling to cooperate with the implementation, so they can understand the needs and concerns of residents through community meetings, questionnaires, etc., and provide detailed answers and explanations. At the same time, residents who have already implemented the optimization measures are invited to share their experiences and feelings to enhance the trust and cooperation of other residents.
Simulation results and analysis before and after optimization
The simulation time is selected from July 19 to 25, 2024 for summer and the winter heating period is from October 1, 2024 to March 31, 2025. The model is shown in Fig. 14, and the indoor thermal environment and winter energy consumption of traditional dwellings before and after the transformation and optimization are compared and analysed.
When simulating the indoor thermal environment, on the simulation output interface, turn off the Heating option to simulate the living room, as shown in Fig. 15. As can be seen from the figure, the optimized enclosure structure enhances the thermal insulation performance, uses materials with low thermal conductivity, etc., reduces the loss of indoor heat, and the indoor temperature of the living room increases every day, and the indoor temperature can be maintained at a high level throughout the day. This effect is especially noticeable in winter, and in other seasons it can also improve living comfort by reducing the temperature difference between day and night. The optimization of the envelope structure is also accompanied by the addition of a waterproof and moisture-proof building layer, which reduces the indoor humidity and combines with the increase in indoor temperature, which is an important reason for the significant increase in the PMV value of the living room. Higher PMV values mean that residents can enjoy a more comfortable indoor environment, contributing to a higher quality of life and satisfaction. The results of this simulation show that by optimizing the envelope, the living room shows a positive effect of increasing the indoor temperature and PMV value in the simulation. These changes not only improve the comfort and quality of occupancy but also help reduce energy consumption and promote sustainable development.
Simulate the energy consumption of the entire building based on winter heating, as shown in Fig. 16. As can be seen from the figure, the heat load in the test period of the model before optimization is 4848.68 kWh, and the maximum heat load occurs in December 2024 and January 2025, which are 1014.93 kWh and 1016.21 kWh, respectively. In the optimized building model, the material changes of the main structure of the exterior wall are simulated and compared, and the thermal parameters of the exterior wall are shown in Table 10: when the aerated concrete material is used, the heat load in the model test period is 2110.84 kW·h, which is compared with the heat load of 4848.68 kW·h before optimization, which significantly reduces the heating energy consumption in winter and saves about 56.5%. When the grass clay is used as the enclosure structure material, the heat load during the model test period is 2150.06 kW·h, and the energy saving is about 55.7%. When stone is used, the heat load during the model test period is 2153.01 kW·h, and the energy saving is about 55.6%. When the tamped clay material is used, the heat load during the model test period is 2155.16 kW·h, and the energy saving is about 55.6%. When light mortar masonry is used to build clay brick masonry, the heat load during the model test period is 2153.49 kW·h, and the energy saving is about 55.6%. The use of different exterior wall main structural materials significantly reduced the heat load during the model test period, and the energy saving rate generally remained at a high level (about 55.6–56.5%), as shown in Fig. 17. This shows that the optimization measures show good robustness under a variety of conditions, which provides strong support for practical engineering applications.
Building orientation
Aba Prefecture is in the western Sichuan Plateau, with less cloud cover and strong solar radiation. The building orientation has a significant impact on the amount of solar radiation received. The orientation of the building model is simulated every 15° from October 1 of 2024 to March 31 of 2025.
The relationship between building orientation and building energy consumption is as follows: when the building orientation is south-west, the heating energy consumption is relatively large, and with the gradual shift of the orientation to the east, the heating energy consumption gradually decreases, and the heating energy consumption reaches a relatively low at 45° south-east. From this angle, the building receives more direct and abundant sunlight for most of the day, which increases the natural temperature of the interior. Therefore, based on the complex relationship between building orientation and solar radiation, indoor thermal environment, and heating energy consumption, the local housing design should try to avoid orientations that are not conducive to energy conservation, such as west orientation. By collating all the simulated data, the relationship between orientation and winter energy consumption can be obtained, and the quantitative relationship can be obtained by linear regression, as shown in Fig. 18.
Analysis of CO2 emissions and economic suitability of heating during Building operation
Cost comparison
-
(a)
Heating methods with electric tools. When calculating the energy saving rate of the envelope structure according to the “Green Performance Calculation Standard for Civil Buildings” JGJ / T449-201855, the annual heating energy consumption during the building operation period of the building should be calculated according to the following formula:
\({{\text{E}}_{h,bld}}=\frac{{{{\text{Q}}_{H,bld}}}}{{{\theta _1}}}\)
Where EH, bld is the annual heating energy consumption of the building; QH, bld is the cumulative heat consumption of the building throughout the year, which is determined by simulation calculation; θ1 is the conversion weight of the comprehensive efficiency of the heating system. According to the optimized simulation calculation, QH, bld =2110.84 kW·h, with the corresponding θ1 = 1.6, then EH, bld =1319.28 kw·h. According to the “Building Carbon Emission Calculation Standard” GB/T51366-201956, Sichuan Province belongs to the Central China regional power grid, and the carbon emission factor f = 0.5257 kg/(kw·h), so the CO2 emissions of heating during operation are EH, bld × f = 1319.28 × 0.5257 = 693.55 kg. Finally, the electricity fee is calculated according to the electricity price, the price of 1 kW·h electricity is between 0.56 ~ 0.62 yuan, calculated at 0.6 yuan/(kw·h), then the annual electricity fee during the operation period Cannual = EH, bld× electricity price = 1319.28 × 0.6 = 791.57 yuan.
-
(b)
Heating methods with household coal. According to the “General Code for Building Energy Conservation and Renewable Energy Utilization” GB55015-2021, the converted weight of the comprehensive efficiency of the heating system is θ1 = 0.88. According to the above formula and simulation calculation, EH, bld = 2398.68 kw·h. The CO2 emission factor per unit calorific value of anthracite coal is 94.44 t/TJ, 1 kW·h = 3.6 × 106 J, 1 TJ = 1012 J, so the carbon emission is 815.51 kg. The coal burning volume of 1 kw·h is equal to 1.229 × 10− 4 t, the coal burning volume during the operation period is 0.29 t, the bituminous coal price is calculated at 510 yuan/t, and the annual coal burning fee during the operation period is 147.9 yuan.
-
(c)
Heating methods with biomass (wood, hay, etc.). According to the General Code for Building Energy Conservation and Renewable Energy Utilization GB55015-2021, the converted weight of the comprehensive efficiency of the heating system is 0.8. According to the simulation results and the above formula, EH, bld=2638.55kw·h, combined with the “Building Carbon Emission Calculation Standard” GB/T51366-2019, the effective CO2 emission factor of wood is 95 t/TJ, so the carbon emission is 902.38 kg. The amount of wood used in 1 kW·h is equal to 4.0 × 10− 4 t, the price of wood is calculated at 839.44 yuan/t, and the timber cost is 889.8 yuan during the annual operation period.
Simulation data and results analysis: From the above simulations, during the operation of the building, the carbon emission is the smallest when the heating mode is electricity, and the carbon emission is the largest when the biomass mode is wood. The use of household coal is the least economical. The carbon emissions simulation data of different heating methods are shown in Fig. 19.
Economic feasibility analysis
-
(a)
Electric heating method. The initial investment cost should consider the purchase and installation costs of electric heating equipment; The known operating cost is 791.57 yuan per year (based on the electricity price of 0.6 yuan/kW·h), and the impact of electricity price fluctuations on long-term operating costs should be considered. The impact of equipment life on long-term maintenance costs should be considered, and the cost of regular maintenance, fault repair, and replacement of parts should be considered; The carbon emission is small, which is conducive to environmental protection and sustainable development.
-
(b)
Household coal heating method. The initial investment cost should consider the purchase and installation of coal-fired stoves and the construction cost of coal storage facilities; Consider the impact of the efficiency and durability of coal-fired stoves on the initial investment; The coal cost is known to be 147.9 yuan per year (based on the coal price of 510 yuan/t), and the impact of coal price fluctuations on operating costs is analysed. Consider the cost of coal storage, transportation, and disposal of cinder; the cost of regular maintenance, breakdown repairs, and replacement of spare parts for coal-fired stoves; Consider the environmental pollution and remediation costs caused by coal burning; Carbon emissions are relatively high, which is not conducive to environmental protection; Consider the potential negative social and economic impacts of environmental pollution.
-
(c)
Biomass (wood, hay, etc.) heating methods. The purchase and installation of biomass combustion equipment and the construction of biomass storage facilities; Consider the impact of the efficiency and durability of biomass burning equipment on the initial investment; The known timber cost is 889.8 yuan per year (based on the timber price of 839.44 yuan/t); Analyse the impact of timber price fluctuations on operating costs; Consider the cost of biomass collection, transportation, and disposal of waste; Biomass, as a renewable energy source, has long-term sustainability; consider the impact of the availability and stability of biomass resources on economic viability; Carbon emissions are high, but there are still certain environmental advantages compared with coal; Consider the specific effects of biomass burning on the environment, such as air quality and ecosystems.
In summary, different heating methods have their advantages and disadvantages in terms of economic viability. In addition to cost comparisons, factors such as initial investment, operating costs, maintenance costs, policy subsidies, tax incentives, environmental benefits, and sustainability should be fully considered during the optimization process.
Return on investment (ROI) and long-term impact
-
(a)
Electric heating. Assuming that the initial investment cost is 5000 yuan, the operating cost of the electric heating mode before optimization is 791.57 yuan/year (based on the electricity price of 0.6 yuan/kW·h). Due to the energy saving of 56.5% after taking optimization measures, the optimized operating cost is 791.57 × (1-56.5%) = 791.57 × 0.435 ≈ 344.42 yuan/year, and the annual saving is 791.57-344.42 = 447.15 yuan/year. The payback period refers to the time from the beginning of the investment to the recovery of the initial investment cost through the saved operating cost, T = 5000/447.15 ≈ 11.18 years. ROI is usually expressed as an annual percentage, \({\text{ROI}} = \frac{{{\text{Annual}}\,{\text{savings}}}}{{{\text{Initial}}\,{\text{investment}}\,{\text{cost}}}} \times 100\% = \frac{{447.15}}{{5000}} \times 100\% = 8.94\%\). Under the assumption that the initial investment cost is 5000 yuan, the return on investment of 56.5% energy saving after optimization is about 8.94%, and the payback period is about 11 years. This means that after about 11 years, the initial investment cost can be recovered by saving operating costs.
-
(b)
Household coal heating. Assuming that the initial investment cost is 2000 yuan, the operating cost of the household coal-fired heating mode before optimization is 147.9 yuan per year (based on the coal price of 510 yuan/t). Due to the energy saving of 56.5% after taking optimization measures, the optimized operating cost is 147.9 × (1-56.5%) = 147.9 × 0.435 ≈ 64.34 yuan/year, and the annual saving is 147.9-64.53 = 83.37 yuan/year. The payback period refers to the time from the beginning of the investment to the recovery of the initial investment cost through the saved operating cost, T = 2000 / 83.37 ≈ 23.99 years. ROI is usually expressed as an annual percentage, \({\text{ROI}} = \frac{{83.37}}{{2000}} \times 100\% = 4.17\%\). Under the assumption that the initial investment cost is 2000 yuan, the return on investment of 56.5% energy saving after optimization of household coal-fired heating mode is about 4.17%, and the payback period is about 24 years. This means that after about 24 years, the initial investment cost can be recovered by saving operating costs.
-
(c)
Biomass heating. Assuming that the initial investment cost is 3000 yuan, the operating cost of the biomass heating method before optimization is 889.8 yuan (based on the wood price of 839.44 yuan/t). Due to the energy saving of 56.5% after taking optimization measures, the optimized operating cost is 889.8 × (1-56.5%) = 889.8 × 0.435 ≈ 387.06 yuan/year, and the annual saving is 889.8-387.06 = 502.74 yuan/year. The payback period refers to the time from the beginning of the investment to the recovery of the initial investment cost through the saved operating cost, T = 3000 / 502.74 ≈ 5.97 years. ROI is usually expressed as an annualized percentage, \({\text{ROI = }}\frac{{502.74}}{{3000}} \times 100\% = 16.77\%\). Assuming that the initial investment cost is 3000 yuan, the return on investment of 56.5% energy saving after optimization of biomass heating mode is about 16.77%, and the payback period is about 6 years. This means that after about 6 years, the initial investment cost can be recovered by saving the operating cost.
In summary, the long-term impact of heating methods involves multiple aspects such as economic, environmental, social and sustainability. When deciding these factors should be considered to choose the heating method that best suits local conditions and needs.